Several methods have been proposed to calculate the CO2Carbon dioxide storage capacityThe accumulated mass of CO2 that can be stored environmentally safely, i.e., without causing leakage of CO2 or native reservoir fluids or triggering geologic activity that has a negative impact on human health or the environment for saline formations. The method used is chosen depending on the available data. Most methods assume that CO2Carbon dioxide storage(CO2) A process for retaining captured CO2, so that it does not reach the atmosphere is achieved by increasing the pressure in the saline formationUnderground rock where saline water occupies the tiny spaces between the grains of rock. Owing to the limited compressibility of fluids and rock, substantial volumes of saline formationUnderground rock where saline water occupies the tiny spaces between the grains of rock are required to maintain useful storage capacityThe accumulated mass of CO2 that can be stored environmentally safely, i.e., without causing leakage of CO2 or native reservoir fluids or triggering geologic activity that has a negative impact on human health or the environment.
When only limited knowledge is available on a potential storage(CO2) A process for retaining captured CO2, so that it does not reach the atmosphere volume, the storage capacityThe accumulated mass of CO2 that can be stored environmentally safely, i.e., without causing leakage of CO2 or native reservoir fluids or triggering geologic activity that has a negative impact on human health or the environment can be estimated from the bulk volume of the saline formationUnderground rock where saline water occupies the tiny spaces between the grains of rock, considering an average porosityMeasure for the amount of pore space in a rock and a storage(CO2) A process for retaining captured CO2, so that it does not reach the atmosphere efficiency factor by multiplication. The bulk formationA body of rock of considerable extent with distinctive characteristics that allow geologists to map, describe, and name it volume is the volume that is hydraulically connected.
The approach that was used to estimate storage capacityThe accumulated mass of CO2 that can be stored environmentally safely, i.e., without causing leakage of CO2 or native reservoir fluids or triggering geologic activity that has a negative impact on human health or the environment in deep saline aquifers in GeoCapacity project was a slightly simplified and/or modified version of the method presented in Bachu et al., 20072007 - S. Bachu, D. Bonijoly, J. Bradshaw, R. Burruss, N. P. Christensen, S. Holloway and O. M. MathiassenEstimation of CO2 Storage Capacity in Geological Media – Phase 2. Work under the auspices of the Carbon Sequestration Leadership Forumsee more. Bachu et al., 20072007 - S. Bachu, D. Bonijoly, J. Bradshaw, R. Burruss, N. P. Christensen, S. Holloway and O. M. MathiassenEstimation of CO2 Storage Capacity in Geological Media – Phase 2. Work under the auspices of the Carbon Sequestration Leadership Forumsee more define both theoretical and effective storage capacityThe accumulated mass of CO2 that can be stored environmentally safely, i.e., without causing leakage of CO2 or native reservoir fluids or triggering geologic activity that has a negative impact on human health or the environment for a basinA geological region with sedimentary strata dipping towards a common axis or centre or region as the sum of the storage capacityThe accumulated mass of CO2 that can be stored environmentally safely, i.e., without causing leakage of CO2 or native reservoir fluids or triggering geologic activity that has a negative impact on human health or the environment of individual structural or stratigraphicThe order and relative position of geological strata Traps in the said area/volume. They then distinguish between theoretical and effective storage capacityThe accumulated mass of CO2 that can be stored environmentally safely, i.e., without causing leakage of CO2 or native reservoir fluids or triggering geologic activity that has a negative impact on human health or the environment by applying a storage(CO2) A process for retaining captured CO2, so that it does not reach the atmosphere efficiency factor (capacity coefficient). The efficiency factor includes the cumulative effects of trap(geology) A geological structure that physically retains fluids that are lighter than the background fluids, e.g. a convex fold heterogeneity, CO2Carbon dioxide buoyancyTendency of a fluid or solid to rise through a fluid of higher density and sweep efficiency; however, the efficiency factor is site-specific and needs to be determined through numerical simulations and/or fieldwork.
An estimate based on the bulk volume of a regional aquiferAn underground layer of fluid-bearing permeable rock or unconsolidated materials (gravel, sand, or silt) with significant permeability to allow flow is, therefore, by nature theoretical. However, theoretical storage capacityThe accumulated mass of CO2 that can be stored environmentally safely, i.e., without causing leakage of CO2 or native reservoir fluids or triggering geologic activity that has a negative impact on human health or the environment estimates are not very useful as they are based on assumptions that are known to be inaccurate. Therefore, applying different storage(CO2) A process for retaining captured CO2, so that it does not reach the atmosphere efficiency factors to the bulk volume of the aquiferAn underground layer of fluid-bearing permeable rock or unconsolidated materials (gravel, sand, or silt) with significant permeability to allow flow is preferred. For bulk volumes of regional aquifers, a storage(CO2) A process for retaining captured CO2, so that it does not reach the atmosphere efficiency factor of 2 % is proposed based on work by the US DOE (Frailey, 20072007 - FraileyEstimation of the Storage Efficiency Factor for Saline Formations. Appendix 1see more). Frailey, 20072007 - FraileyEstimation of the Storage Efficiency Factor for Saline Formations. Appendix 1see more found values ranging between 1.8 and 2.2 % for the storage(CO2) A process for retaining captured CO2, so that it does not reach the atmosphere efficiency factor of the bulk volume of a regional aquiferAn underground layer of fluid-bearing permeable rock or unconsolidated materials (gravel, sand, or silt) with significant permeability to allow flow through Monte CarloA modelling technique in which the statistical properties of outcomes are tested by random inputs simulations (with low and high values of 1 % and 4 %, respectively).
Capacity estimation standards based on the work and publications of the CSLF (Bachu et al., 20072007 - S. Bachu, D. Bonijoly, J. Bradshaw, R. Burruss, N. P. Christensen, S. Holloway and O. M. MathiassenEstimation of CO2 Storage Capacity in Geological Media – Phase 2. Work under the auspices of the Carbon Sequestration Leadership Forumsee more) do not provide advice on the value of trap(geology) A geological structure that physically retains fluids that are lighter than the background fluids, e.g. a convex fold specific storage(CO2) A process for retaining captured CO2, so that it does not reach the atmosphere efficiency factor, other than it is very much site-specific. Discussions on storage(CO2) A process for retaining captured CO2, so that it does not reach the atmosphere efficiency factors in several research projects have led to further work on this issue by the IEAInternational Energy Agency and the CSLF. In the EUEuropean Union GeoCapacity project, two different approaches were developed for trap(geology) A geological structure that physically retains fluids that are lighter than the background fluids, e.g. a convex fold-specific storage(CO2) A process for retaining captured CO2, so that it does not reach the atmosphere efficiency factors, one for open or semi-closed aquiferAn underground layer of fluid-bearing permeable rock or unconsolidated materials (gravel, sand, or silt) with significant permeability to allow flow systems and one for closed aquiferAn underground layer of fluid-bearing permeable rock or unconsolidated materials (gravel, sand, or silt) with significant permeability to allow flow systems.
Bachu et al., 20072007 - S. Bachu, D. Bonijoly, J. Bradshaw, R. Burruss, N. P. Christensen, S. Holloway and O. M. MathiassenEstimation of CO2 Storage Capacity in Geological Media – Phase 2. Work under the auspices of the Carbon Sequestration Leadership Forumsee more include the net to gross ratio (NG) in both theoretical and the effective capacity estimates, which is meaningful when assessing individual traps. The net to gross ratio is, however, also a site specific parameter, which depends on the local geological conditions and is not necessarily wellManmade hole drilled into the earth to produce liquids or gases, or to allow the injection of fluids known or homogeneously distributed throughout a region. It may, therefore, not be meaningful to establish an average value for a regional aquiferAn underground layer of fluid-bearing permeable rock or unconsolidated materials (gravel, sand, or silt) with significant permeability to allow flow based on few observations. If limited information is available, a default value of 0.25 is suggested. This value may be too high in some cases, but will for many cases be a conservative value. When considering the NG ratio, it should normally be possible to provide a best estimate of the reservoirA subsurface body of rock with sufficient porosity and permeability to store and transmit fluids porosityMeasure for the amount of pore space in a rock of a regional aquiferAn underground layer of fluid-bearing permeable rock or unconsolidated materials (gravel, sand, or silt) with significant permeability to allow flow.
CO2Carbon dioxide density is a function of pressure and temperature and can be obtained from different models (e.g, Span and Wagner, 19961996 - R. Span and W. WagnerA New Equation of State for Carbon Dioxide covering the Fluid Region from the Triple-point Temperature to 1100 K at Pressures up to 800 MPasee more and Peneloux et al., 19821982 - A. Peneloux, E. Rauzy and R. FrezeA Consistent Correction for Redlich-Kwong-Soave Volumessee more). Again, it may not be meaningful to establish an average value for a regional aquiferAn underground layer of fluid-bearing permeable rock or unconsolidated materials (gravel, sand, or silt) with significant permeability to allow flow based on insufficient observations.
As a regional estimate based on bulk volume of an aquiferAn underground layer of fluid-bearing permeable rock or unconsolidated materials (gravel, sand, or silt) with significant permeability to allow flow rather than trap(geology) A geological structure that physically retains fluids that are lighter than the background fluids, e.g. a convex fold volumes is already subject to great uncertainty (thickness and extent of aquiferAn underground layer of fluid-bearing permeable rock or unconsolidated materials (gravel, sand, or silt) with significant permeability to allow flow, storage(CO2) A process for retaining captured CO2, so that it does not reach the atmosphere efficiency factor, etc.), the exact values of the net to gross ratio and the CO2Carbon dioxide density are not essential. Furthermore, as the value of the storage(CO2) A process for retaining captured CO2, so that it does not reach the atmosphere efficiency factor is generalised rather than based on specific geological conditions, a regional estimate calculated using this methodology should be regarded as only indicative.
When information to estimate the pressure increase that can be applied to the hydraulically connected volume is available, a more reliable estimate of the storage capacityThe accumulated mass of CO2 that can be stored environmentally safely, i.e., without causing leakage of CO2 or native reservoir fluids or triggering geologic activity that has a negative impact on human health or the environment of a saline formationUnderground rock where saline water occupies the tiny spaces between the grains of rock can be obtained. combined with the compressibility of the fluids and rock, the storage capacityThe accumulated mass of CO2 that can be stored environmentally safely, i.e., without causing leakage of CO2 or native reservoir fluids or triggering geologic activity that has a negative impact on human health or the environment estimate can be derived using the following equation (see, e.g. Frailey, 20072007 - FraileyEstimation of the Storage Efficiency Factor for Saline Formations. Appendix 1see more).
MCO2b = A × h × NG × f × rCO2Carbon dioxider × Dp × (br+bf)
where in addition to the parameters defined in Tab. 2-4, Dp is the pressures increase (relative to the initial pressure), br is the compressibility of the matrix; bf is compressibility of the fluid.
Tab. 2-4: Parameters used in the static capacity assessment of saline aquifers (Vangkilde-Pedersen et al., 2008). Parameter | MCO2Carbon dioxide | A | h | NG | φ | ρCO2r | Seff | Description | Regional or trap(geology) A geological structure(geology) Geological feature produced by the deformation of the Earth’s crust, such as a fold or a fault(geology) A surface at which strata are no longer continuous, but are found displaced; a feature within a rock such as a fractureAny break in rock along which no significant movement has occurred; or, more generally, the spatial arrangement of rocks that physically retains fluids that are lighter than the background fluids, e.g. a convex fold aquiferAn underground layer of fluid-bearing permeable rock or unconsolidated materials (gravel, sand, or silt) with significant permeability to allow flow storage(CO2Carbon dioxide) A process for retaining captured CO2Carbon dioxide, so that it does not reach the atmosphereThe layer of gases surrounding the earth; the gases are mainly nitrogen (78%) and oxygen (around 21%) capacityThe accumulated mass of CO2Carbon dioxide that can be stored environmentally safely, i.e., without causing leakage(in CO2Carbon dioxide storage) The escape of injected fluid from the storage formationA body of rock of considerable extent with distinctive characteristics that allow geologists to map, describe, and name it to the atmosphereThe layer of gases surrounding the earth; the gases are mainly nitrogen (78%) and oxygen (around 21%) or water column of CO2Carbon dioxide or native reservoirA subsurface body of rock with sufficient porosityMeasure for the amount of pore spaceSpace between rock or sediment grains that can contain fluids in a rock and permeabilityAbility to flow or transmit fluids through a porous solid such as rock to store and transmit fluids fluids or triggering geologic activity that has a negative impact on human health or the environment | Area of aquiferAn underground layer of fluid-bearing permeable rock or unconsolidated materials (gravel, sand, or silt) with significant permeability to allow flow | Average thickness of aquiferAn underground layer of fluid-bearing permeable rock or unconsolidated materials (gravel, sand, or silt) with significant permeability to allow flow | Average net to gross ratio of aquiferAn underground layer of fluid-bearing permeable rock or unconsolidated materials (gravel, sand, or silt) with significant permeability to allow flow | Average reservoirA subsurface body of rock with sufficient porosityMeasure for the amount of pore spaceSpace between rock or sediment grains that can contain fluids in a rock and permeabilityAbility to flow or transmit fluids through a porous solid such as rock to store and transmit fluids porosityMeasure for the amount of pore spaceSpace between rock or sediment grains that can contain fluids in a rock | CO2Carbon dioxide density at reservoirA subsurface body of rock with sufficient porosityMeasure for the amount of pore spaceSpace between rock or sediment grains that can contain fluids in a rock and permeabilityAbility to flow or transmit fluids through a porous solid such as rock to store and transmit fluids conditions | Storage(CO2Carbon dioxide) A process for retaining captured CO2Carbon dioxide, so that it does not reach the atmosphereThe layer of gases surrounding the earth; the gases are mainly nitrogen (78%) and oxygen (around 21%) efficiency factor | Typical values | Gtonnes to Mtonnes | Thousands to tens of km2 | Hundreds of meters | Tens of percent | 10-30% | 0.6-0.8 g/cm3 | 2-3% (regional)* 10-40% (trap(geology) A geological structure(geology) Geological feature produced by the deformation of the Earth’s crust, such as a fold or a fault(geology) A surface at which strata are no longer continuous, but are found displaced; a feature within a rock such as a fractureAny break in rock along which no significant movement has occurred; or, more generally, the spatial arrangement of rocks that physically retains fluids that are lighter than the background fluids, e.g. a convex fold - closed to fully open)* | *Also reservoirA subsurface body of rock with sufficient porosityMeasure for the amount of pore spaceSpace between rock or sediment grains that can contain fluids in a rock and permeabilityAbility to flow or transmit fluids through a porous solid such as rock to store and transmit fluids properties matter (if better, the coefficient is higher); if Seff=0, then MCO2Carbon dioxide is simply a theoretical capacity. |
If the knowledge and level of detail exists and regional capacity estimates are available, it is more reliable to provide storage capacityThe accumulated mass of CO2 that can be stored environmentally safely, i.e., without causing leakage of CO2 or native reservoir fluids or triggering geologic activity that has a negative impact on human health or the environment estimates that take into account the volume in traps, where the buoyant CO2Carbon dioxide can be safely retained. It should be emphasised here that storage capacityThe accumulated mass of CO2 that can be stored environmentally safely, i.e., without causing leakage of CO2 or native reservoir fluids or triggering geologic activity that has a negative impact on human health or the environment in saline formations is not only limited by the pressure increase that can be sustained by the formationA body of rock of considerable extent with distinctive characteristics that allow geologists to map, describe, and name it, but also by the traps where CO2Carbon dioxide collects after injectionThe process of using pressure to force fluids down wells. The volume of CO2Carbon dioxide that is derived from the connected volume and assumed pressure increase must be stored in an open structure(geology) Geological feature produced by the deformation of the Earth’s crust, such as a fold or a fault; a feature within a rock such as a fracture; or, more generally, the spatial arrangement of rocks that will retain the CO2Carbon dioxide. The pressure in this volume can be increased to create space for CO2Carbon dioxide within the structure(geology) Geological feature produced by the deformation of the Earth’s crust, such as a fold or a fault; a feature within a rock such as a fracture; or, more generally, the spatial arrangement of rocks. The volume of CO2Carbon dioxide that can be trapped is defined by the volume in the traps to its spill pointThe structurally lowest point in a structural trap that can retain fluids lighter than background fluids. The smaller of these two volumes (CO2Carbon dioxide volume from pressure increase, trap(geology) A geological structure that physically retains fluids that are lighter than the background fluids, e.g. a convex fold volume) defines the total storage(CO2) A process for retaining captured CO2, so that it does not reach the atmosphere volume.
The most accurate storage capacityThe accumulated mass of CO2 that can be stored environmentally safely, i.e., without causing leakage of CO2 or native reservoir fluids or triggering geologic activity that has a negative impact on human health or the environment estimate is obtained after a detailed site characterisation study. In such a study, all available data is collected, a detailed geological model of the connected volume is created and a reservoirA subsurface body of rock with sufficient porosity and permeability to store and transmit fluids engineering study is performed to obtain a realistic storage(CO2) A process for retaining captured CO2, so that it does not reach the atmosphere and injectionThe process of using pressure to force fluids down wells capacity estimate.
Storage capacityThe accumulated mass of CO2 that can be stored environmentally safely, i.e., without causing leakage of CO2 or native reservoir fluids or triggering geologic activity that has a negative impact on human health or the environment estimations for saline aquiferAn underground layer of fluid-bearing permeable rock or unconsolidated materials (gravel, sand, or silt) with significant permeability to allow flow Reservoirs are often presented in the form of a storage(CO2) A process for retaining captured CO2, so that it does not reach the atmosphere pyramid (Bachu, 20032003 - S. BachuScreening and ranking sedimentary basins for sequestration of CO2 in geological media in response to climate changesee more; Kaldi and Gibson-Poole, 20082008 - J. G. Kaldi and C. M. Gibson-PooleStorage Capacity Estimation, Site Selection and Characterisation for CO2 Storage Projectssee more; Vangkilde-Pedersen et al., 2008). Fig. 2-5 and 2-6 present two such examples. Depending on whether the detailed structure(geology) Geological feature produced by the deformation of the Earth’s crust, such as a fold or a fault; a feature within a rock such as a fracture; or, more generally, the spatial arrangement of rocks geometry and reservoirA subsurface body of rock with sufficient porosity and permeability to store and transmit fluids parameters are known, assessment of the realistic capacity might require new geophysical surveys and wellManmade hole drilled into the earth to produce liquids or gases, or to allow the injection of fluids drilling. It should be noted that, in any case, reliable economic evaluation cannot be completed without acquisition of new geological data.
Generally, for matched (or practical) capacity an injectionThe process of using pressure to force fluids down wells scenarioA plausible description of the future based on an internally consistent set of assumptions about key relationships and driving forces; note that scenarios are neither predictions nor forecasts and simulations are necessary (discussed in the following sections); while realistic (or effective) capacity, as a minimum, can be assessed using a robust static capacity estimation approach (Vangkilde-Pedersen et al., 2008).
For this approach, based on calculation of pore spaceSpace between rock or sediment grains that can contain fluids volume available for injectionThe process of using pressure to force fluids down wells and storage(CO2) A process for retaining captured CO2, so that it does not reach the atmosphere, the following formula on static capacity assessment has been recommended in the EUEuropean Union GeoCapacity project (Vangkilde-Pedersen et al., 2008; Tab. 2-4) after CSLF guidelines (Bachu et al., 20072007 - S. Bachu, D. Bonijoly, J. Bradshaw, R. Burruss, N. P. Christensen, S. Holloway and O. M. MathiassenEstimation of CO2 Storage Capacity in Geological Media – Phase 2. Work under the auspices of the Carbon Sequestration Leadership Forumsee more):
MCO2Carbon dioxide = A × h × NG × φ × ρCO2r × Seff
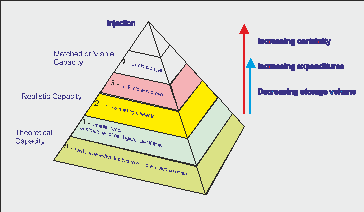 Fig. 2-5: Storage(CO2Carbon dioxide) A process for retaining captured CO2Carbon dioxide, so that it does not reach the atmosphereThe layer of gases surrounding the earth; the gases are mainly nitrogen (78%) and oxygen (around 21%) capacityThe accumulated mass of CO2Carbon dioxide that can be stored environmentally safely, i.e., without causing leakage(in CO2Carbon dioxide storage) The escape of injected fluid from the storage formationA body of rock of considerable extent with distinctive characteristics that allow geologists to map, describe, and name it to the atmosphereThe layer of gases surrounding the earth; the gases are mainly nitrogen (78%) and oxygen (around 21%) or water column of CO2Carbon dioxide or native reservoirA subsurface body of rock with sufficient porosityMeasure for the amount of pore spaceSpace between rock or sediment grains that can contain fluids in a rock and permeabilityAbility to flow or transmit fluids through a porous solid such as rock to store and transmit fluids fluids or triggering geologic activity that has a negative impact on human health or the environment pyramid for saline aquifers (modified after Bachu, 20032003 - S. BachuScreening and ranking sedimentary basins for sequestration of CO2 in geological media in response to climate changesee more). |
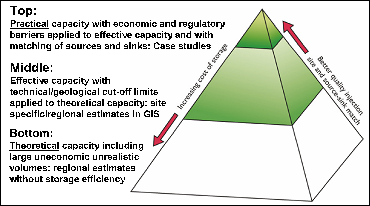 Fig. 2-6: A simplified storage(CO2Carbon dioxide) A process for retaining captured CO2Carbon dioxide, so that it does not reach the atmosphereThe layer of gases surrounding the earth; the gases are mainly nitrogen (78%) and oxygen (around 21%) capacityThe accumulated mass of CO2Carbon dioxide that can be stored environmentally safely, i.e., without causing leakage(in CO2Carbon dioxide storage) The escape of injected fluid from the storage formationA body of rock of considerable extent with distinctive characteristics that allow geologists to map, describe, and name it to the atmosphereThe layer of gases surrounding the earth; the gases are mainly nitrogen (78%) and oxygen (around 21%) or water column of CO2Carbon dioxide or native reservoirA subsurface body of rock with sufficient porosityMeasure for the amount of pore spaceSpace between rock or sediment grains that can contain fluids in a rock and permeabilityAbility to flow or transmit fluids through a porous solid such as rock to store and transmit fluids fluids or triggering geologic activity that has a negative impact on human health or the environment pyramid for saline aquifers (Vangkilde-Pedersen et al., 2008). |