The Sleipner CO2Carbon dioxide storage(CO2Carbon dioxide) A process for retaining captured CO2Carbon dioxide, so that it does not reach the atmosphereThe layer of gases surrounding the earth; the gases are mainly nitrogen (78%) and oxygen (around 21%) project is the world's largest and longest running Carbon CaptureThe separation of carbon dioxide from other gases before it is emitted to the atmosphereThe layer of gases surrounding the earth; the gases are mainly nitrogen (78%) and oxygen (around 21%) and Storage(CO2Carbon dioxide) A process for retaining captured CO2Carbon dioxide, so that it does not reach the atmosphereThe layer of gases surrounding the earth; the gases are mainly nitrogen (78%) and oxygen (around 21%) (CCSCarbon dioxide CaptureThe separation of carbon dioxide from other gases before it is emitted to the atmosphereThe layer of gases surrounding the earth; the gases are mainly nitrogen (78%) and oxygen (around 21%) and Storage) project. The Sleipner fields are situated in the Norwegian North Sea. They produce gas with a high CO2Carbon dioxide content from Jurassic and TertiaryGeological age from about 65 to 2 million years ago reservoirs. The CO2Carbon dioxide is separated from the hydrocarbons at the Sleipner T platform and, since 1996, 13 million tonnes of CO2Carbon dioxide have been injected into the Utsira FormationA body of rock of considerable extent with distinctive characteristics that allow geologists to map, describe, and name it of Miocene age. This formationA body of rock of considerable extent with distinctive characteristics that allow geologists to map, describe, and name it consists of up to 300 m thick sandstones with 90 - 98 % sand content, with average porosityMeasure for the amount of pore spaceSpace between rock or sediment grains that can contain fluids in a rock of 35 - 40 %, net/gross ratio of 0.90 - 0.97, and permeabilities in the 1-8 Darcies range. The subsurface CO2Carbon dioxide plumeDispersing volume of CO2Carbon dioxide-rich phase contained in target formationA body of rock of considerable extent with distinctive characteristics that allow geologists to map, describe, and name it has been monitored from the surface by six time-lapse seismic surveys. Chadwick et al., 20092009 - A. Chadwick, D. Noy, R. J. Arts and O. EikenLatest time-lapse seismic data from Sleipner yield new insights into CO2 plume developmentsee more presented seismic images of the CO2Carbon dioxide plumeDispersing volume of CO2Carbon dioxide-rich phase contained in target formationA body of rock of considerable extent with distinctive characteristics that allow geologists to map, describe, and name it at Sleipner showing its development up to 2006 (Fig. 3-4). The first repeat seismic survey (1999) revealed that migrating CO2Carbon dioxide had spread to nine distinct layers - one of these lying above a 5 - 6.5 m thick shaleClay that has changed into a rock due to geological processes. The migrating CO2Carbon dioxide cumulating under the low-permeabilityAbility to flow or transmit fluids through a porous solid such as rock layers appears to have been fed from a central vertical feeder, which is referred as a seismic chimney in the seismic data. (Alnes et al., 20112011 - H. Alnes, O. Eiken, S. Nooner, G. Sasagawa, T. Stenvold and M. ZumbergeResults from Sleipner gravity monitoring: Updated density and temperature distribution of the CO2 plumesee more; Hermanrud et al., 20092009 - C. Hermanrud, T. Andresen, O. Eiken, H. Hansen, A. Janbu, J. Lippard and H. N. BolåsStorage of CO2 in saline aquifers - Lessons learned from 10 years of injection into the Utsira Formation in the Sleipner areasee more).
Fig. 3-4: Seismic images of the Sleipner plume showing its development to 2006. Top) N-S seismic section through the plume. Topmost CO2Carbon dioxide layer arrowed. Bottom) plan views of the plume showing total integrated reflection amplitude (Chadwick et al., 20092009 - A. Chadwick, D. Noy, R. J. Arts and O. EikenLatest time-lapse seismic data from Sleipner yield new insights into CO2 plume developmentsee more). |
Instead of a complex numerical approach, Bickle et al., 2007 studied CO2Carbon dioxide migrationThe movement of fluids in reservoir rocks and accumulation at the Sleipner storage(CO2Carbon dioxide) A process for retaining captured CO2Carbon dioxide, so that it does not reach the atmosphereThe layer of gases surrounding the earth; the gases are mainly nitrogen (78%) and oxygen (around 21%) site in the North Sea using modified wellManmade hole drilled into the earth to produce liquids or gases, or to allow the injectionThe process of using pressure to force fluids down wells of fluids-known solutions for (axisymmetric) gravity flows within a permeable medium. The results indicate that CO2Carbon dioxide accumulation under the shallower low-permeabilityAbility to flow or transmit fluids through a porous solid such as rock layers within the reservoirA subsurface body of rock with sufficient porosityMeasure for the amount of pore spaceSpace between rock or sediment grains that can contain fluids in a rock and permeabilityAbility to flow or transmit fluids through a porous solid such as rock to store and transmit fluids started sometime after the start of injectionThe process of using pressure to force fluids down wells of CO2Carbon dioxide in the field. Modelling of the time variation of thickness in two of these layers indicated that their CO2Carbon dioxide input increased with time. Conversely, under the deeper layers net CO2Carbon dioxide inputs appear to decrease with time. It seems most probable that the deeper layers progressively leaked more CO2Carbon dioxide through their thin caprockRock of very low permeability that acts as an upper seal to prevent fluid flow out of a reservoir mudstones with time and the growth of the overlying layers reflects this increasing supply of CO2Carbon dioxide from below.
The reactive transport modelling study conducted by BRGM (Picot-Colbeaux et. al., 20092009 - G. Picot-Colbeaux, F. Mathurin and P. AudiganeShort-term reactive multi- phase flow modeling for performance assessment of CO2 storage at Sleipnersee more) using TOUGHREACT showed that after three years of injectionThe process of using pressure to force fluids down wells, the primary trapping(CO2Carbon dioxide) ContainmentRestriction of the movement of a fluid to a designated volume (e.g. reservoir) or immobilisation of CO2Carbon dioxide, there are four main trapping mechanisms: structural or stratigraphicThe order and relative position of geological strata trapping; residual CO2Carbon dioxide trapping (capillary trappingImmobilisation of a fraction of in-situ fluids by capillary forces) by capillary forces; solubility trappingA process in which fluids are retained by dissolution in liquids naturally present by dissolution of CO2Carbon dioxide in resident formationA body of rock of considerable extent with distinctive characteristics that allow geologists to map, describe, and name it fluids forming a non-buoyant fluid; and mineral trapping where CO2Carbon dioxide is absorbed by solid minerals present in the storage(CO2Carbon dioxide) A process for retaining captured CO2Carbon dioxide, so that it does not reach the atmosphereThe layer of gases surrounding the earth; the gases are mainly nitrogen (78%) and oxygen (around 21%) volume process is the geological structure(geology) Geological feature produced by the deformation of the Earth’s crust, such as a fold or a fault(geology) A surface at which strata are no longer continuous, but are found displaced; a feature within a rock such as a fractureAny break in rock along which no significant movement has occurred; or, more generally, the spatial arrangement of rocks of the system. The upward migrationThe movement of fluids in reservoir rocks of the free CO2Carbon dioxide due to buoyancyTendency of a fluid or solid to rise through a fluid of higher density forces is prevented by the low-permeabilityAbility to flow or transmit fluids through a porous solid such as rock shaleClay that has changed into a rock due to geological processes layers but mainly by the caprockRock of very low permeability that acts as an upper seal to prevent fluid flow out of a reservoir formationA body of rock of considerable extent with distinctive characteristics that allow geologists to map, describe, and name it overlying the reservoirA subsurface body of rock with sufficient porosityMeasure for the amount of pore spaceSpace between rock or sediment grains that can contain fluids in a rock and permeabilityAbility to flow or transmit fluids through a porous solid such as rock to store and transmit fluids aquiferAn underground layer of fluid-bearing permeable rock or unconsolidated materials (gravel, sand, or silt) with significant permeability to allow flow. The dissolution mechanism is also modelled and it was seen that although the solubilisation had started, it did not represent more than 10% of the injected CO2Carbon dioxide amount (Audigane et al., 20112011 - P. Audigane, C. Chiaberge, F. Mathurin, J. Lions and G. Picot-ColbeauxA workflow for handling heterogeneous 3D models with the TOUGH2 family of codes Applications to numerical modeling of CO2 geological storagesee more).
One of the studies to understand plume evolution and storage(CO2Carbon dioxide) A process for retaining captured CO2Carbon dioxide, so that it does not reach the atmosphereThe layer of gases surrounding the earth; the gases are mainly nitrogen (78%) and oxygen (around 21%) performance is performed by Chadwick and Noy, 20102010 - A. Chadwick and D. J. NoyHistory-matching flow simulations and time-lapse seismic data from the Sleipner CO2 plume, Geological Society, Londonsee more. The need for the adjustments on the capillary entry pressureAdditional pressure needed for a liquid or gas to enter a pore and overcome surface tension and the permeabilityAbility to flow or transmit fluids through a porous solid such as rock of the intra-reservoirA subsurface body of rock with sufficient porosityMeasure for the amount of pore spaceSpace between rock or sediment grains that can contain fluids in a rock and permeabilityAbility to flow or transmit fluids through a porous solid such as rock to store and transmit fluids mudstoneA very fine-grained sedimentary rock formed from mud in a 2D THOUGH2 flow model (Fig. 3-5) in order to match the observed arrival time of CO2Carbon dioxide at top of the reservoirA subsurface body of rock with sufficient porosityMeasure for the amount of pore spaceSpace between rock or sediment grains that can contain fluids in a rock and permeabilityAbility to flow or transmit fluids through a porous solid such as rock to store and transmit fluids, showed that CO2Carbon dioxide migrationThe movement of fluids in reservoir rocks through the mudstones is actually not by Darcy flow but via some form of pathway flow, possibly associated with networks of small faults or perhaps holes. According to the 3D THOUGH2 flow model, the increased CO2Carbon dioxide fluxes to the topmost layer suggest that the feeder pathways are evolving, becoming either more transmissive with time and/or increasing in number. The history match of the lateral spreading rates could be achieved by using very high anisotropic permeabilities as can be seen in Fig. 3-6.
Fig. 3-5: Simulated growth of the CO2Carbon dioxide plumeDispersing volume of CO2Carbon dioxide-rich phase contained in target formationA body of rock of considerable extent with distinctive characteristics that allow geologists to map, describe, and name it from 1999 to 2006 from the THOUGH2 axisymmetrical flow model (Chadwick and Noy, 20102010 - A. Chadwick and D. J. NoyHistory-matching flow simulations and time-lapse seismic data from the Sleipner CO2 plume, Geological Society, Londonsee more). |
MigrationThe movement of fluids in reservoir rocks of CO2Carbon dioxide through permeable pathways, formed by overlapping turbidite lobes or channels, in otherwise sealing cap-rocks has been modelled by Grimstad et al., 20092009 - A. A. Grimstad, S. Georgescu, E. Lindeberg and J. F. VuillaumeModelling and Simulation of Mechanisms for Leakage of CO2 from Geological Storagesee more. The simulation results are then fitted using an analytical expression. It was concluded that the longer it takes the CO2Carbon dioxide to migrate to the surface, the larger the lateral spread of the CO2Carbon dioxide plumeDispersing volume of CO2Carbon dioxide-rich phase contained in target formationA body of rock of considerable extent with distinctive characteristics that allow geologists to map, describe, and name it would be.
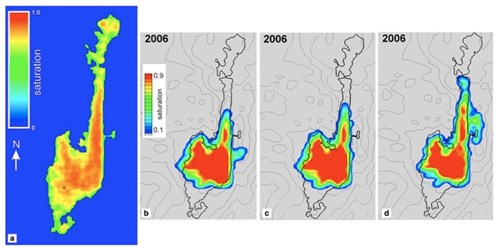 Fig. 3-6: The topmost layer in 2006. a) observed extents; b) THOUGH2 simulation with k=6 Darcy; c) THOUGH2 simulation with k=3 Darcy east-west and 10 Darcy north-south; d) THOUGH2 simulation with k=3 Darcy east-west, 10 Darcy north-south and higher reservoirA subsurface body of rock with sufficient porosityMeasure for the amount of pore spaceSpace between rock or sediment grains that can contain fluids in a rock and permeabilityAbility to flow or transmit fluids through a porous solid such as rock to store and transmit fluids temperature (Chadwick and Noy, 20102010 - A. Chadwick and D. J. NoyHistory-matching flow simulations and time-lapse seismic data from the Sleipner CO2 plume, Geological Society, Londonsee more). |