Xiao et al., 20092009 - Y. Xiao, T. Xu and K. PruessThe effects of gas-fluid-rock interactions on CO2 injection and storage: insights from reactive transport modellingsee more summarised the advances in reactive transport modelling of CO2Carbon dioxide storage(CO2) A process for retaining captured CO2, so that it does not reach the atmosphere and reviewed the key technical issues on; (1) the short- and long-term behaviour of CO2Carbon dioxide injected in geological formations; (2) the role of reservoirA subsurface body of rock with sufficient porosity and permeability to store and transmit fluids mineral heterogeneity on injectionThe process of using pressure to force fluids down wells performance and storage(CO2) A process for retaining captured CO2, so that it does not reach the atmosphere security; (3) the effect of gas mixtures (e.g. H2S and SO2) on CO2Carbon dioxide storage(CO2) A process for retaining captured CO2, so that it does not reach the atmosphere; and (4) the physical and chemical processes acting in case of CO2Carbon dioxide leakage(in CO2 storage) The escape of injected fluid from the storage formation to the atmosphere or water column from the primary storage(CO2) A process for retaining captured CO2, so that it does not reach the atmosphere reservoirA subsurface body of rock with sufficient porosity and permeability to store and transmit fluids.
Using the TOUGHREACT reactive transport modelling code, Xiao et al., 20092009 - Y. Xiao, T. Xu and K. PruessThe effects of gas-fluid-rock interactions on CO2 injection and storage: insights from reactive transport modellingsee more investigated mixed CO2Carbon dioxide/H2S/SO2 injectionThe process of using pressure to force fluids down wells and storage(CO2) A process for retaining captured CO2, so that it does not reach the atmosphere in both siliciclastic and carbonateNatural minerals (e.g. calcite, dolomite, siderite, limestone) composed of various anions bonded to a CO32- cation reservoirs for a 1D radial reactive transport model design simulating CO2Carbon dioxide injectionThe process of using pressure to force fluids down wells 2 km depth and 70oC temperature in a siliciclastic and carbonateNatural minerals (e.g. calcite, dolomite, siderite, limestone) composed of various anions bonded to a CO32- cation reservoirA subsurface body of rock with sufficient porosity and permeability to store and transmit fluids. CO2Carbon dioxide and other gases were injected into the reservoirA subsurface body of rock with sufficient porosity and permeability to store and transmit fluids at a rate of 1 million tonnes per year over a 100 years period. The reactive transport models simulate the system from 0 to 10,000 years. Three scenarios of mixed gas injections were selected: (a) CO2Carbon dioxide only, (b) CO2Carbon dioxide + H2S, and (c) CO2Carbon dioxide + SO2, in which CO2Carbon dioxide is injected as gas phase while both H2S and SO2 (~5% each) are injected as aqueous solutes. The Reservoirs are specified to have an initial porosityMeasure for the amount of pore space in a rock of 30% and an initial permeabilityAbility to flow or transmit fluids through a porous solid such as rock of 100 mDA non-SI unit of permeability, milli Darcy, and approximately equal to 1μm2. The siliciclastic and carbonateNatural minerals (e.g. calcite, dolomite, siderite, limestone) composed of various anions bonded to a CO32- cation reservoirs were defined by hypothetical mineral assemblages, representing an oligoclase feldsparA group of alumino-silicate minerals (K, Na)AlSi3O8 - CaAl2Si2O8 that makes up much of the Earth’s crust-rich sandstoneSand that has turned into a rock due to geological processes reservoirA subsurface body of rock with sufficient porosity and permeability to store and transmit fluids and a limestoneA sedimentary rock made mostly of the mineral calcite (calcium carbonate), usually formed from shells of dead organisms-rich reservoirA subsurface body of rock with sufficient porosity and permeability to store and transmit fluids (Xiao et al., 2009).
Simulations provided estimates of pH evolution, mineral dissolution/precipitation and CO2Carbon dioxide storage(CO2) A process for retaining captured CO2, so that it does not reach the atmosphere for sandstoneSand that has turned into a rock due to geological processes reservoirA subsurface body of rock with sufficient porosity and permeability to store and transmit fluids Injections and pH evolution and mineral dissolution/precipitation for carbonateNatural minerals (e.g. calcite, dolomite, siderite, limestone) composed of various anions bonded to a CO32- cation reservoirA subsurface body of rock with sufficient porosity and permeability to store and transmit fluids injectionThe process of using pressure to force fluids down wells. The evolution of porosityMeasure for the amount of pore space in a rock was modelled for both Reservoirs (Fig. 4-2 and 4-3). In the siliciclastic reservoirA subsurface body of rock with sufficient porosity and permeability to store and transmit fluids, whatever the injected gas is (CO2Carbon dioxide only, CO2Carbon dioxide + H2S, and CO2Carbon dioxide + SO2), injectionThe process of using pressure to force fluids down wells induces an increase of porosityMeasure for the amount of pore space in a rock (that is much more important in case of CO2Carbon dioxide + SO2) close to the wellManmade hole drilled into the earth to produce liquids or gases, or to allow the injection of fluids due to net mineral dissolution, and a decrease away from the wellManmade hole drilled into the earth to produce liquids or gases, or to allow the injection of fluids due to mineral trapping(CO2) Containment or immobilisation of CO2, there are four main trapping mechanisms: structural or stratigraphic trapping; residual CO2 trapping (capillary trapping) by capillary forces; solubility trapping by dissolution of CO2 in resident formation fluids forming a non-buoyant fluid; and mineral trapping where CO2 is absorbed by solid minerals present in the storage volume in all three cases (Xiao et al., 2009). In the carbonateNatural minerals (e.g. calcite, dolomite, siderite, limestone) composed of various anions bonded to a CO32- cation reservoirA subsurface body of rock with sufficient porosity and permeability to store and transmit fluids, a significant increase of the porosityMeasure for the amount of pore space in a rock (30% to 40% after 100 years) is observed when CO2Carbon dioxide or CO2Carbon dioxide + H2S is injected due to calcite dissolution near the wellManmade hole drilled into the earth to produce liquids or gases, or to allow the injection of fluids bore. These results indicate that there is little mineral trapping(CO2) Containment or immobilisation of CO2, there are four main trapping mechanisms: structural or stratigraphic trapping; residual CO2 trapping (capillary trapping) by capillary forces; solubility trapping by dissolution of CO2 in resident formation fluids forming a non-buoyant fluid; and mineral trapping where CO2 is absorbed by solid minerals present in the storage volume in all three mixed gas injectionThe process of using pressure to force fluids down wells scenarios, suggesting limited CO2Carbon dioxide storage capacityThe accumulated mass of CO2 that can be stored environmentally safely, i.e., without causing leakage of CO2 or native reservoir fluids or triggering geologic activity that has a negative impact on human health or the environment in a limestoneA sedimentary rock made mostly of the mineral calcite (calcium carbonate), usually formed from shells of dead organisms dominated carbonateNatural minerals (e.g. calcite, dolomite, siderite, limestone) composed of various anions bonded to a CO32- cation reservoirA subsurface body of rock with sufficient porosity and permeability to store and transmit fluids. Xiao et al., 20092009 - Y. Xiao, T. Xu and K. PruessThe effects of gas-fluid-rock interactions on CO2 injection and storage: insights from reactive transport modellingsee more concludes that reactive transport modelling provides valuable insights for describing, analysing, interpreting, and assessing the physical properties and dynamic behaviours of injected CO2Carbon dioxide as wellManmade hole drilled into the earth to produce liquids or gases, or to allow the injection of fluids as for facilitating the screening and evaluation of CO2Carbon dioxide storage(CO2) A process for retaining captured CO2, so that it does not reach the atmosphere strategy.
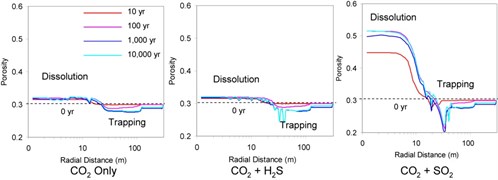 Fig. 4-2: PorosityMeasure for the amount of pore spaceSpace between rock or sediment grains that can contain fluids in a rock evolution as a function of radial distance simulated at 10, 100, 1,000, and 10,000 years for the mixed gas injectionThe process of using pressure to force fluids down wells Scenarios in a siliciclastic reservoirA subsurface body of rock with sufficient porosityMeasure for the amount of pore spaceSpace between rock or sediment grains that can contain fluids in a rock and permeabilityAbility to flow or transmit fluids through a porous solid such as rock to store and transmit fluids (Xiao et al., 20092009 - Y. Xiao, T. Xu and K. PruessThe effects of gas-fluid-rock interactions on CO2 injection and storage: insights from reactive transport modellingsee more). |
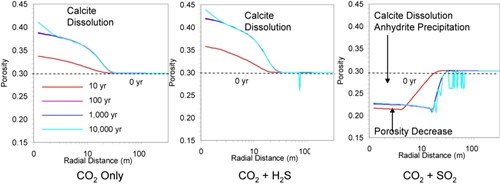 Fig. 4-3: PorosityMeasure for the amount of pore spaceSpace between rock or sediment grains that can contain fluids in a rock evolution as a function of radial distance simulated at 10, 100, 1,000, and 10,000 years for three mixed gas injectionThe process of using pressure to force fluids down wells Scenarios in a carbonateNatural minerals (e.g. calcite, dolomite, siderite, limestone) composed of various anions bonded to a CO32- cation reservoirA subsurface body of rock with sufficient porosityMeasure for the amount of pore spaceSpace between rock or sediment grains that can contain fluids in a rock and permeabilityAbility to flow or transmit fluids through a porous solid such as rock to store and transmit fluids (Xiao et al., 20092009 - Y. Xiao, T. Xu and K. PruessThe effects of gas-fluid-rock interactions on CO2 injection and storage: insights from reactive transport modellingsee more) |