Faults represent an important geological feature significantly influencing the CO2Carbon dioxide storage(CO2) A process for retaining captured CO2, so that it does not reach the atmosphere complex. Their role with respect to the storage(CO2) A process for retaining captured CO2, so that it does not reach the atmosphere reservoirA subsurface body of rock with sufficient porosity and permeability to store and transmit fluids can be twofold:
- Permeable faults serve as preferred migrationThe movement of fluids in reservoir rocks routes for both the injected CO2Carbon dioxide and the original reservoirA subsurface body of rock with sufficient porosityMeasure for the amount of pore spaceSpace between rock or sediment grains that can contain fluids in a rock and permeabilityAbility to flow or transmit fluids through a porous solid such as rock to store and transmit fluids fluids. In case they intersect the impermeable caprockRock of very low permeability that acts as an upper seal to prevent fluid flow out of a reservoir, they may become migrationThe movement of fluids in reservoir rocks pathways leading to leakage(in CO2Carbon dioxide storage) The escape of injected fluid from the storage formationA body of rock of considerable extent with distinctive characteristics that allow geologists to map, describe, and name it to the atmosphereThe layer of gases surrounding the earth; the gases are mainly nitrogen (78%) and oxygen (around 21%) or water column of CO2Carbon dioxide out of the reservoirA subsurface body of rock with sufficient porosityMeasure for the amount of pore spaceSpace between rock or sediment grains that can contain fluids in a rock and permeabilityAbility to flow or transmit fluids through a porous solid such as rock to store and transmit fluids.
- Impermeable faults act as barriers for migrationThe movement of fluids in reservoir rocks of CO2Carbon dioxide and reservoirA subsurface body of rock with sufficient porosityMeasure for the amount of pore spaceSpace between rock or sediment grains that can contain fluids in a rock and permeabilityAbility to flow or transmit fluids through a porous solid such as rock to store and transmit fluids fluids, often causing reservoirA subsurface body of rock with sufficient porosityMeasure for the amount of pore spaceSpace between rock or sediment grains that can contain fluids in a rock and permeabilityAbility to flow or transmit fluids through a porous solid such as rock to store and transmit fluids compartmentalisation. Consequences of this situation might be, e.g. reduced storage(CO2Carbon dioxide) A process for retaining captured CO2Carbon dioxide, so that it does not reach the atmosphereThe layer of gases surrounding the earth; the gases are mainly nitrogen (78%) and oxygen (around 21%) capacityThe accumulated mass of CO2Carbon dioxide that can be stored environmentally safely, i.e., without causing leakage(in CO2Carbon dioxide storage) The escape of injected fluid from the storage formationA body of rock of considerable extent with distinctive characteristics that allow geologists to map, describe, and name it to the atmosphereThe layer of gases surrounding the earth; the gases are mainly nitrogen (78%) and oxygen (around 21%) or water column of CO2Carbon dioxide or native reservoirA subsurface body of rock with sufficient porosityMeasure for the amount of pore spaceSpace between rock or sediment grains that can contain fluids in a rock and permeabilityAbility to flow or transmit fluids through a porous solid such as rock to store and transmit fluids fluids or triggering geologic activity that has a negative impact on human health or the environment and/or injectivityA measure of the rate at which a quantity of fluid can be injected into a well of the reservoirA subsurface body of rock with sufficient porosityMeasure for the amount of pore spaceSpace between rock or sediment grains that can contain fluids in a rock and permeabilityAbility to flow or transmit fluids through a porous solid such as rock to store and transmit fluids, rapid pressure increase around the injectionThe process of using pressure to force fluids down wells wellManmade hole drilled into the earth to produce liquids or gases, or to allow the injectionThe process of using pressure to force fluids down wells of fluids.
Determination of exact location and geometry of faults in the storage(CO2) A process for retaining captured CO2, so that it does not reach the atmosphere complex and investigation of their character belong to the most important goals of site characterisation. Usually, the first information on fault(geology) A surface at which strata are no longer continuous, but are found displaced presence and geometry as wellManmade hole drilled into the earth to produce liquids or gases, or to allow the injection of fluids as their first-order characterisation are obtained from a 3D seismic survey. To assess the large-scale heterogeneity of the storage(CO2) A process for retaining captured CO2, so that it does not reach the atmosphere container, including its vertical and lateral compartmentalisation and the sealing nature of fractures and faults, pressure measurements may be employed, e.g. during wellManmade hole drilled into the earth to produce liquids or gases, or to allow the injection of fluids-tests or by Repeat FormationA body of rock of considerable extent with distinctive characteristics that allow geologists to map, describe, and name it Tester (RFT) or Modular FormationA body of rock of considerable extent with distinctive characteristics that allow geologists to map, describe, and name it Dynamics Tester (MDT) logging (Arts et al., 20092009 - R. Arts, van den Belt F., Benedictus T., Blom M., A. de Buck, H. Croezen, C. Hofstee, Ch. Kolesar, T. Koryl, M. Lewitt, M. Nepveu, H. Stoiber, S. Wartmann, T. WildenborgSupport to the Introduction of the Enabling Legal Framework on Carbon Dioxide Capture and Storage (CCS)see more).
Mapping of faults from seismic data and building a fault(geology) A surface at which strata are no longer continuous, but are found displaced model is an important activity when creating the three-dimensional static geological earth model of the storage(CO2) A process for retaining captured CO2, so that it does not reach the atmosphere complex. This commonly involves simplification or generalisation of fault(geology) A surface at which strata are no longer continuous, but are found displaced planes. This initial fault(geology) A surface at which strata are no longer continuous, but are found displaced model is usually refined by wellManmade hole drilled into the earth to produce liquids or gases, or to allow the injection of fluids correlation when sub-seismic faults may become apparent, especially in cases when stratigraphicThe order and relative position of geological strata sections are missing (normal faults) or doubled (reverse faults).
It is important to assess the sealing capacity of faults as this may lead to compartmentalisation of the storage(CO2) A process for retaining captured CO2, so that it does not reach the atmosphere reservoirA subsurface body of rock with sufficient porosity and permeability to store and transmit fluids and/or leakage(in CO2 storage) The escape of injected fluid from the storage formation to the atmosphere or water column through the caprockRock of very low permeability that acts as an upper seal to prevent fluid flow out of a reservoir. compartmentalisation is a key input in the earth model, as it will lead to rapid pressure increase and demands for a relatively high number of injectionThe process of using pressure to force fluids down wells wells (Arts et al., 20092009 - R. Arts, van den Belt F., Benedictus T., Blom M., A. de Buck, H. Croezen, C. Hofstee, Ch. Kolesar, T. Koryl, M. Lewitt, M. Nepveu, H. Stoiber, S. Wartmann, T. WildenborgSupport to the Introduction of the Enabling Legal Framework on Carbon Dioxide Capture and Storage (CCS)see more). At the same time, knowledge of fault(geology) A surface at which strata are no longer continuous, but are found displaced properties is also critical for geomechanical modelling of the reservoirA subsurface body of rock with sufficient porosity and permeability to store and transmit fluids.
Fault(geology) A surface at which strata are no longer continuous, but are found displaced properties are also important for geochemical characterisation of the site and related geochemical modelling. The nature of fault(geology) A surface at which strata are no longer continuous, but are found displaced-filling minerals must be assessed, since the sealing potential of faults cutting the reservoirA subsurface body of rock with sufficient porosity and permeability to store and transmit fluids or overburdenRocks and sediments above any particular stratum may be reduced as a result of CO2Carbon dioxide induced dissolution. To avoid the riskConcept that denotes the product of the probability of a hazard and the subsequent consequence of the associated event of decreasing sealing potential in response to dissolution of carbonates, the carbonateNatural minerals (e.g. calcite, dolomite, siderite, limestone) composed of various anions bonded to a CO32- cation content of sealing formations should be accurately studied and incorporated in the earth model, in particular if these formations are fractured or faulted (Arts et al., 20092009 - R. Arts, van den Belt F., Benedictus T., Blom M., A. de Buck, H. Croezen, C. Hofstee, Ch. Kolesar, T. Koryl, M. Lewitt, M. Nepveu, H. Stoiber, S. Wartmann, T. WildenborgSupport to the Introduction of the Enabling Legal Framework on Carbon Dioxide Capture and Storage (CCS)see more).
MonitoringMeasurement and surveillance activities necessary for ensuring safe and reliable operation of a CGS project (storage integrity), and for estimating emission reductions of faults
MonitoringMeasurement and surveillance activities necessary for ensuring safe and reliable operation of a CGS project (storage integrity), and for estimating emission reductions of faults can be divided into two main issues:
- monitoringMeasurement and surveillance activities necessary for ensuring safe and reliable operation of a CGS project (storage integrity), and for estimating emission reductions of changes in the fault(geology) A surface at which strata are no longer continuous, but are found displaced system in and around the reservoirA subsurface body of rock with sufficient porosityMeasure for the amount of pore spaceSpace between rock or sediment grains that can contain fluids in a rock and permeabilityAbility to flow or transmit fluids through a porous solid such as rock to store and transmit fluids, which especially comprises changes in the integrity and sealing properties of the faults;
- monitoringMeasurement and surveillance activities necessary for ensuring safe and reliable operation of a CGS project (storage integrity), and for estimating emission reductions of potential leakages of CO2Carbon dioxide from the storage(CO2Carbon dioxide) A process for retaining captured CO2Carbon dioxide, so that it does not reach the atmosphereThe layer of gases surrounding the earth; the gases are mainly nitrogen (78%) and oxygen (around 21%) reservoirA subsurface body of rock with sufficient porosityMeasure for the amount of pore spaceSpace between rock or sediment grains that can contain fluids in a rock and permeabilityAbility to flow or transmit fluids through a porous solid such as rock to store and transmit fluids via faults as preferential migrationThe movement of fluids in reservoir rocks pathways.
The integrity of faults is part of the storage(CO2) A process for retaining captured CO2, so that it does not reach the atmosphere reservoirA subsurface body of rock with sufficient porosity and permeability to store and transmit fluids integrity as a whole, and the monitoringMeasurement and surveillance activities necessary for ensuring safe and reliable operation of a CGS project (storage integrity), and for estimating emission reductions methods for these purposes are wellManmade hole drilled into the earth to produce liquids or gases, or to allow the injection of fluids established. Usually, the first indicator of a change in the reservoirA subsurface body of rock with sufficient porosity and permeability to store and transmit fluids behaviour is a sudden change in reservoirA subsurface body of rock with sufficient porosity and permeability to store and transmit fluids pressure.
Such a change needs to be detected as soon as possible since it normally indicates an unpredicted, and often undesired, event in the reservoirA subsurface body of rock with sufficient porosity and permeability to store and transmit fluids, which may (in the worst case) correspond to a significant irregularityAny irregularity in the injection or storage operation or in the condition of the storage volume itself, which implies the risk of a leakage or risk to the environment or human health according to the EU CCS DirectiveDirective 2009/31/EC of the European Parliament and of the Council of 23 April 2009 on the geological storage of carbon dioxide or even to a CO2Carbon dioxide leakage(in CO2 storage) The escape of injected fluid from the storage formation to the atmosphere or water column from the reservoirA subsurface body of rock with sufficient porosity and permeability to store and transmit fluids. Such a pressure change can have various causes, a change in fault(geology) A surface at which strata are no longer continuous, but are found displaced integrity being one of them. Alternatively, the change in fault(geology) A surface at which strata are no longer continuous, but are found displaced properties can be a slow process with slower changes in reservoirA subsurface body of rock with sufficient porosity and permeability to store and transmit fluids pressure. In both cases, however, reliable continuous reservoirA subsurface body of rock with sufficient porosity and permeability to store and transmit fluids pressure measurement is the main condition for timely detection of the change.
Permanent measurements of reservoirA subsurface body of rock with sufficient porosity and permeability to store and transmit fluids pressure are part of commonly used, permanent downhole monitoringMeasurement and surveillance activities necessary for ensuring safe and reliable operation of a CGS project (storage integrity), and for estimating emission reductions technologies that have been used in the oil and gas industry for several decades. Measurement devices and downhole installation services are commercially available. The gauges are usually installed in the wellManmade hole drilled into the earth to produce liquids or gases, or to allow the injection of fluids casingA pipe which is inserted to stabilise the borehole of a well after it is drilled to be able to read the formationA body of rock of considerable extent with distinctive characteristics that allow geologists to map, describe, and name it pressure directly (see Fig. 2-14). The gauges are normally installed in monitoringMeasurement and surveillance activities necessary for ensuring safe and reliable operation of a CGS project (storage integrity), and for estimating emission reductions wells penetrating the storage(CO2) A process for retaining captured CO2, so that it does not reach the atmosphere formationA body of rock of considerable extent with distinctive characteristics that allow geologists to map, describe, and name it but they can also be placed in adjacent overburdenRocks and sediments above any particular stratum layers, preferably on places where presence of faults has been detected or assumed. An example of a pressure monitoringMeasurement and surveillance activities necessary for ensuring safe and reliable operation of a CGS project (storage integrity), and for estimating emission reductions record is shown in Fig. 2-15.
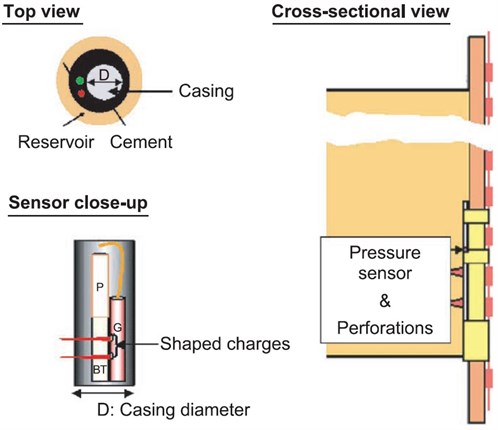 Fig. 2-14: Graphical illustration of a permanent downhole pressure gauge installation. The gauge is cemented behind casingA pipe which is inserted to stabilise the borehole of a well after it is drilled and operates in direct hydraulic communication with the formationA body of rock of considerable extent with distinctive characteristics that allow geologists to map, describe, and name it (Alpak et al., 20042004 - Faruk O Alpak, Carlos Torres-Verdı́n, Kamy SepehrnooriEstimation of axisymmetric spatial distributions of permeability and porosity from pressure-transient data acquired with in situ permanent sensorssee more). |
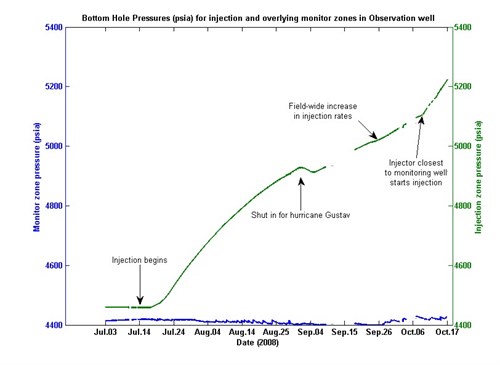 Fig. 2-15: Example of reservoirA subsurface body of rock with sufficient porosityMeasure for the amount of pore spaceSpace between rock or sediment grains that can contain fluids in a rock and permeabilityAbility to flow or transmit fluids through a porous solid such as rock to store and transmit fluids pressure monitoringMeasurement and surveillance activities necessary for ensuring safe and reliable operation of a CGS project (storage integrity), and for estimating emission reductions at the Cranfield CO2Carbon dioxide-EOREnhanced Oil RecoveryThe recovery of oil additional to that produced naturally, achieved by fluid injection or other means: the recovery of oil additional to that produced naturally, achieved by fluid injection or other means site in Mississippi, USA. The plot shows evolution of pressure within injectionThe process of using pressure to force fluids down wells zone (green) and overlying monitoringMeasurement and surveillance activities necessary for ensuring safe and reliable operation of a CGS project (storage integrity), and for estimating emission reductions zone (blue). Pressure in the injectionThe process of using pressure to force fluids down wells zone has significantly increased since injectionThe process of using pressure to force fluids down wells start, while monitoringMeasurement and surveillance activities necessary for ensuring safe and reliable operation of a CGS project (storage integrity), and for estimating emission reductions zone pressure has stayed constant, indicating no communication between the two zones. Annotations are for various events during field injectionThe process of using pressure to force fluids down wells. Data gaps are a result of data communication issues between downhole gauges and surface recording devices (Meckel et al., 20082008 - TA Meckel, SD Hovorka, N KalyanaramanContinuous pressure monitoring for large volume CO2 injectionssee more). |
Another technique able to detect changes in fault(geology) A surface at which strata are no longer continuous, but are found displaced integrity is passive seismic monitoringMeasurement and surveillance activities necessary for ensuring safe and reliable operation of a CGS project (storage integrity), and for estimating emission reductions. Re-activation of existing faults or formationA body of rock of considerable extent with distinctive characteristics that allow geologists to map, describe, and name it of new faults and fractures caused by increased reservoirA subsurface body of rock with sufficient porosityMeasure for the amount of pore spaceSpace between rock or sediment grains that can contain fluids in a rock and permeabilityAbility to flow or transmit fluids through a porous solid such as rock to store and transmit fluids pressure produce seismic activity that may be monitored in the form of microseismic or even seismic events by passive seismic monitoringMeasurement and surveillance activities necessary for ensuring safe and reliable operation of a CGS project (storage integrity), and for estimating emission reductions (see example in Fig. 2-16). For more details regarding this technique see Section 2.3.
Changes in fault(geology) A surface at which strata are no longer continuous, but are found displaced extent, shape and properties can also be derived from time-lapse measurements of various kinds that are part of the site monitoringMeasurement and surveillance activities necessary for ensuring safe and reliable operation of a CGS project (storage integrity), and for estimating emission reductions plan. This is especially valid for 3D seismics, wellManmade hole drilled into the earth to produce liquids or gases, or to allow the injectionThe process of using pressure to force fluids down wells of fluids-logging in monitoringMeasurement and surveillance activities necessary for ensuring safe and reliable operation of a CGS project (storage integrity), and for estimating emission reductions wells or surface deformation monitoringMeasurement and surveillance activities necessary for ensuring safe and reliable operation of a CGS project (storage integrity), and for estimating emission reductions. The results of time-lapse measurements may lead to updates in both reservoirA subsurface body of rock with sufficient porosityMeasure for the amount of pore spaceSpace between rock or sediment grains that can contain fluids in a rock and permeabilityAbility to flow or transmit fluids through a porous solid such as rock to store and transmit fluids and geomechanical models and simulations, including the characteristics and role of the fault(geology) A surface at which strata are no longer continuous, but are found displaced system (see also Sections 2.1 and 2.2).
Methods for monitoringMeasurement and surveillance activities necessary for ensuring safe and reliable operation of a CGS project (storage integrity), and for estimating emission reductions of potential leakages of CO2Carbon dioxide from the reservoirA subsurface body of rock with sufficient porosityMeasure for the amount of pore spaceSpace between rock or sediment grains that can contain fluids in a rock and permeabilityAbility to flow or transmit fluids through a porous solid such as rock to store and transmit fluids via permeable faults are more or less identical with the methods suitable for monitoringMeasurement and surveillance activities necessary for ensuring safe and reliable operation of a CGS project (storage integrity), and for estimating emission reductions of overlying and adjacent aquifers (Section 2.6), freshwater aquifers (Section 2.7) and near surface eco-compartments (Section 2.8). The known presence of faults leads to a special areal or spatial focus of monitoringMeasurement and surveillance activities necessary for ensuring safe and reliable operation of a CGS project (storage integrity), and for estimating emission reductions layouts that can be concentrated to places/areas above or close to existing faults to allow early detection of a potential leakage(in CO2Carbon dioxide storage) The escape of injected fluid from the storage formationA body of rock of considerable extent with distinctive characteristics that allow geologists to map, describe, and name it to the atmosphereThe layer of gases surrounding the earth; the gases are mainly nitrogen (78%) and oxygen (around 21%) or water column.
These monitoringMeasurement and surveillance activities necessary for ensuring safe and reliable operation of a CGS project (storage integrity), and for estimating emission reductions targets correspond to the segments of the subsurface where the CO2Carbon dioxide migrating from the storage(CO2Carbon dioxide) A process for retaining captured CO2Carbon dioxide, so that it does not reach the atmosphereThe layer of gases surrounding the earth; the gases are mainly nitrogen (78%) and oxygen (around 21%) reservoirA subsurface body of rock with sufficient porosityMeasure for the amount of pore spaceSpace between rock or sediment grains that can contain fluids in a rock and permeabilityAbility to flow or transmit fluids through a porous solid such as rock to store and transmit fluids can accumulate. The NETL Best Practices manual (2009) mentions two techniques particularly suitable for monitoringMeasurement and surveillance activities necessary for ensuring safe and reliable operation of a CGS project (storage integrity), and for estimating emission reductions for leakage(in CO2Carbon dioxide storage) The escape of injected fluid from the storage formationA body of rock of considerable extent with distinctive characteristics that allow geologists to map, describe, and name it to the atmosphereThe layer of gases surrounding the earth; the gases are mainly nitrogen (78%) and oxygen (around 21%) or water column into overlying formations through faults or fractures:
- pressure monitoringMeasurement and surveillance activities necessary for ensuring safe and reliable operation of a CGS project (storage integrity), and for estimating emission reductions in the overlying formationA body of rock of considerable extent with distinctive characteristics that allow geologists to map, describe, and name it (see Fig. 2-14 for example), and
- monitoringMeasurement and surveillance activities necessary for ensuring safe and reliable operation of a CGS project (storage integrity), and for estimating emission reductions for tracers (e.g. PFCs).
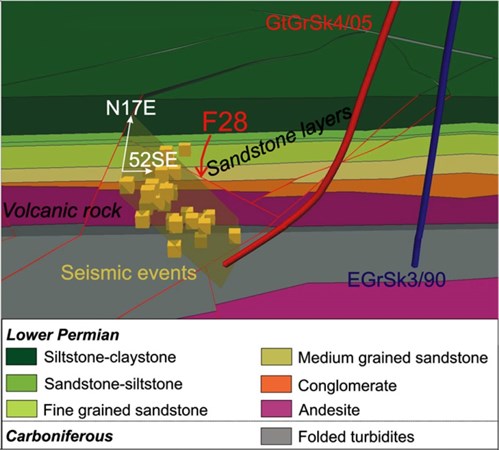 Fig. 2-16: Interpretation of microseismic events recorded during water injectionThe process of using pressure to force fluids down wells operations at the Groß Schönebeck geothermalConcerning heat flowing from deep in the earth research field, Germany. Yellow boxes show interpreted locations of low-magnitude microseismic events, probably occurring along an existing fault(geology) A surface at which strata are no longer continuous, but are found displaced plane and indicating thus reactivation of an existing fault(geology) A surface at which strata are no longer continuous, but are found displaced. The additional fluid pressure was between 20 and 24.5 MPa (Moeck et al., 20092009 - Inga Moeck, Grzegorz Kwiatek, Günter ZimmermannSlip tendency analysis, fault reactivation potential and induced seismicity in a deep geothermal reservoirsee more). Similar events may potentially happen at CO2Carbon dioxide storage(CO2Carbon dioxide) A process for retaining captured CO2Carbon dioxide, so that it does not reach the atmosphereThe layer of gases surrounding the earth; the gases are mainly nitrogen (78%) and oxygen (around 21%) sites, if the reservoirA subsurface body of rock with sufficient porosityMeasure for the amount of pore spaceSpace between rock or sediment grains that can contain fluids in a rock and permeabilityAbility to flow or transmit fluids through a porous solid such as rock to store and transmit fluids pressure exceeds safety pressure limits. |
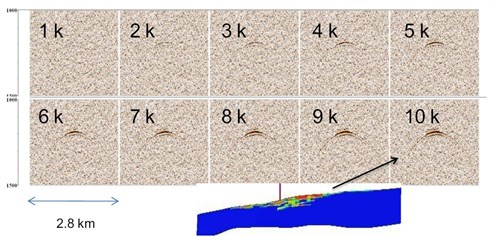 Fig. 2-17: Results of seismic 4D modelling of the "leak" scenarioA plausible description of the future based on an internally consistent set of assumptions about key relationships and driving forces; note that scenarios are neither predictions nor forecasts at the Otway basinA geological region with sedimentary strata dipping towards a common axis or centre Pilot Project in Australia. In the modelled case, CO2Carbon dioxide migrates from the Naylor reservoirA subsurface body of rock with sufficient porosityMeasure for the amount of pore spaceSpace between rock or sediment grains that can contain fluids in a rock and permeabilityAbility to flow or transmit fluids through a porous solid such as rock to store and transmit fluids along the Naylor fault(geology) A surface at which strata are no longer continuous, but are found displaced into the overlying Paaratte formationA body of rock of considerable extent with distinctive characteristics that allow geologists to map, describe, and name it. Distribution of CO2Carbon dioxide for the model was extracted from reservoirA subsurface body of rock with sufficient porosityMeasure for the amount of pore spaceSpace between rock or sediment grains that can contain fluids in a rock and permeabilityAbility to flow or transmit fluids through a porous solid such as rock to store and transmit fluids simulation for various leakage(in CO2Carbon dioxide storage) The escape of injected fluid from the storage formationA body of rock of considerable extent with distinctive characteristics that allow geologists to map, describe, and name it to the atmosphereThe layer of gases surrounding the earth; the gases are mainly nitrogen (78%) and oxygen (around 21%) or water column quantities. The leaked CO2Carbon dioxide shows up as diffraction, in this case submerged into the background noise proportional in magnitude and frequency content to the actual one observed during field experiments (Urosevic et al., 20112011 - M. Urosevic, R. Pevzner, V. Shulakova, A. Kepic, E. Caspari, S. SharmaSeismic monitoring of CO2 injection into a depleted gas reservoir–Otway Basin Pilot Project, Australiasee more). |
Time-lapse measurements represent another group of techniques potentially capable of detecting leaking faults. Urosevic et al., 20112011 - M. Urosevic, R. Pevzner, V. Shulakova, A. Kepic, E. Caspari, S. SharmaSeismic monitoring of CO2 injection into a depleted gas reservoir–Otway Basin Pilot Project, Australiasee more modelled 4D seismic response of CO2Carbon dioxide leaking along a fault(geology) A surface at which strata are no longer continuous, but are found displaced from the Naylor reservoirA subsurface body of rock with sufficient porosityMeasure for the amount of pore spaceSpace between rock or sediment grains that can contain fluids in a rock and permeabilityAbility to flow or transmit fluids through a porous solid such as rock to store and transmit fluids within the Otway basinA geological region with sedimentary strata dipping towards a common axis or centre Pilot Project in Australia. The authors demonstrated that small quantities of CO2Carbon dioxide are likely to produce very strong changes in the elastic properties of the host rock(geology) The rock formationA body of rock of considerable extent with distinctive characteristics that allow geologists to map, describe, and name it that contains a foreign material that would be readily detected by time lapse seismic monitoringMeasurement and surveillance activities necessary for ensuring safe and reliable operation of a CGS project (storage integrity), and for estimating emission reductions (see Fig. 2-17).