Induced seismicityThe episodic occurrence of natural or man-induced earthquakes may be related to the injectionThe process of using pressure to force fluids down wells of CO2Carbon dioxide into deep aquifers (Sminchak et al., 20022002 - Joel Sminchak, Neeraj Gupta, C Byrer, P BergmanIssues related to seismic activity induced by the injection of CO2 in deep saline aquiferssee more) and (depleted(hydrocarbon reservoir) one where production is significantly reduced) hydrocarbon fields. Generally, induced seismicityThe episodic occurrence of natural or man-induced earthquakes has long been recognised as a part of human activities such as oil and gas production, dam building, geothermalConcerning heat flowing from deep in the earth energy production, mining, quarrying and underground gas storage(CO2) A process for retaining captured CO2, so that it does not reach the atmosphere. The study of induced seismicityThe episodic occurrence of natural or man-induced earthquakes has been going on for more than 50 years with two main drivers: (a) the riskConcept that denotes the product of the probability of a hazard and the subsequent consequence of the associated event, damage and public concern caused by ground motion and (b) the potential to monitor subsurface processes via the induced seismicityThe episodic occurrence of natural or man-induced earthquakes. Therefore, induced seismicityThe episodic occurrence of natural or man-induced earthquakes is recognised as a potential issue affecting geological storage(CO2) A process for retaining captured CO2, so that it does not reach the atmosphere of CO2Carbon dioxide, both as a hazard and as a reservoirA subsurface body of rock with sufficient porosity and permeability to store and transmit fluids monitoringMeasurement and surveillance activities necessary for ensuring safe and reliable operation of a CGS project (storage integrity), and for estimating emission reductions tool. Regarding the potential hazard, there is a significant technical knowledge base referring to seismicityThe episodic occurrence of natural or man-induced earthquakes induced from human activities. One important distinction can be made between 'triggered' seismicityThe episodic occurrence of natural or man-induced earthquakes and true 'induced' seismicityThe episodic occurrence of natural or man-induced earthquakes. 'Triggered' seismicityThe episodic occurrence of natural or man-induced earthquakes includes those events that would have occurred naturally at some point in the future but were triggered by human activity, while 'induced' seismicityThe episodic occurrence of natural or man-induced earthquakes comprises those events entirely caused by human activity. It is important for CO2Carbon dioxide storage(CO2) A process for retaining captured CO2, so that it does not reach the atmosphere projects to develop a uniform general approach to the induced seismicityThe episodic occurrence of natural or man-induced earthquakes hazard. Additional investigations will be needed to improve the understanding and estimation of the potential induced seismicityThe episodic occurrence of natural or man-induced earthquakes hazard at any individual site (Myer and Daley, 20112011 - Larry R. Myer, Thomas M. DaleyElements of a best practices approach to induced seismicity in geologic storagesee more). These investigations may include a structural study of the area, historical seismicityThe episodic occurrence of natural or man-induced earthquakes, evaluation of the critical fluid pressure for failure and pre-injectionThe process of using pressure to force fluids down wells seismic monitoringMeasurement and surveillance activities necessary for ensuring safe and reliable operation of a CGS project (storage integrity), and for estimating emission reductions of the area to define "zero-state" seismicityThe episodic occurrence of natural or man-induced earthquakes (Holloway, 20012001 - Sam HollowayStorage of fossil fuel-derived carbon dioxide beneath the surface of the earthsee more; Chang, 20072007 - W.K. ChangA simulation study of injected CO2 migration in the faulted reservoirsee more).
InjectionThe process of using pressure to force fluids down wells of CO2Carbon dioxide into porous rocks at pressures higher than formationA body of rock of considerable extent with distinctive characteristics that allow geologists to map, describe, and name it pressures can induce fracturing and fault(geology) A surface at which strata are no longer continuous, but are found displaced activation. This may pose two kinds of risks (Benson et al., 20052005 - S. Benson, P. Cook, J. Anderson, S. Bachu, H. Bashir Nimir, B. Basu, J. Bradshaw, G. Deguchi, J. Gale, G. Goerne, W. Heidug, S. Holloway, R. Kamal, D. Keith, P. Lloyd, P. Rocha, B. Senior, J. Thomson, T. Torp, T. Wildenborg, M. Wilson, F. Zarlenga, D. Zhou, M. Celia, Gunter B., J. Ennis King, E. Lindeberg, S. Lombardi, C. Oldenburg, K. Pruess, A. Rigg, S. Stevens, E. Wilson, S. Whittaker, G. Borm, D. Hawkins, A. LeeUnderground geological storage. In: Carbon Dioxide Capture and Storagesee more):
- brittle failure and associated microseismicitySmall-scale seismic tremors induced by overpressurisation can create or enhance fractureAny break in rock along which no significant movement has occurred permeabilityAbility to flow or transmit fluids through a porous solid such as rock (secondary permeabilityAbility to flow or transmit fluids through a porous solid such as rock), thus providing pathways for unwanted CO2Carbon dioxide migrationThe movement of fluids in reservoir rocks.
- fault(geology) A surface at which strata are no longer continuous, but are found displaced activation may induce earthquakes.
An understanding of the structural geology, lithologyThe nature and composition of rocks and hydrology of the CO2Carbon dioxide storage(CO2) A process for retaining captured CO2, so that it does not reach the atmosphere site is critical to determining if injectionThe process of using pressure to force fluids down wells will induce seismic events (Sminchak et al., 20022002 - Joel Sminchak, Neeraj Gupta, C Byrer, P BergmanIssues related to seismic activity induced by the injection of CO2 in deep saline aquiferssee more).
Mechanisms and processes
A conceptual model of possible processes potentially involved in triggering seismic activity by underground injectionThe process of using pressure to force fluids down wells wells is given in Fig. 2-10.
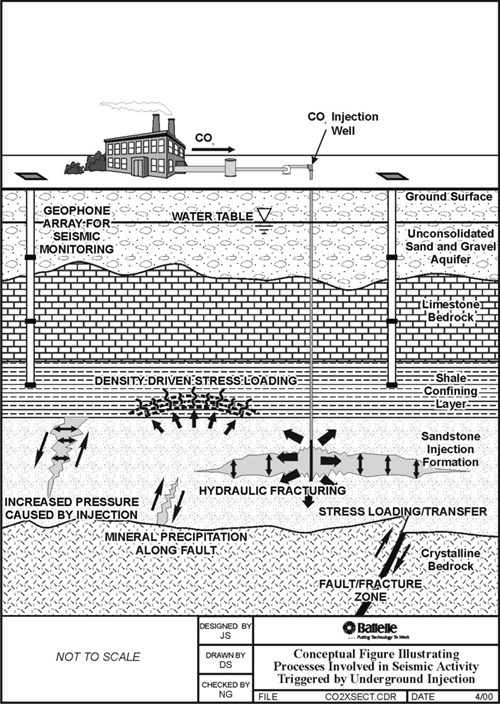 Fig. 2-10: Conceptual figure illustrating potential processes involved in seismic activity possibly induced by underground injectionThe process of using pressure to force fluids down wells wells (Sminchak et al., 20022002 - Joel Sminchak, Neeraj Gupta, C Byrer, P BergmanIssues related to seismic activity induced by the injection of CO2 in deep saline aquiferssee more). |
During the process of CO2Carbon dioxide injectionThe process of using pressure to force fluids down wells at a storage(CO2) A process for retaining captured CO2, so that it does not reach the atmosphere site, in-situ stresses will be modified by pore pressure increases, creating a potential for seismic events due to slippage upon pre-existing discontinuities or due to creation of new fractures (Myer and Daley, 20112011 - Larry R. Myer, Thomas M. DaleyElements of a best practices approach to induced seismicity in geologic storagesee more). The greatest riskConcept that denotes the product of the probability of a hazard and the subsequent consequence of the associated event for induced seismicityThe episodic occurrence of natural or man-induced earthquakes will probably arise from slip on pre-existing faults and fractures. Other processes involved in the triggering of seismic activity may include transfer of stress to a weaker fault(geology) A surface at which strata are no longer continuous, but are found displaced, hydraulic fractureAny break in rock along which no significant movement has occurred, mineral precipitation along a fault(geology) A surface at which strata are no longer continuous, but are found displaced, density-driven stress loading etc. Processes involving faults are described and discussed in more detail in Section 2.4.
In terms of stress equation, deep wellManmade hole drilled into the earth to produce liquids or gases, or to allow the injection of fluids injectionThe process of using pressure to force fluids down wells reduces both the principal and confining pressure in the injectionThe process of using pressure to force fluids down wells formationA body of rock of considerable extent with distinctive characteristics that allow geologists to map, describe, and name it while keeping the differential pressure constant, moving the system toward failure (cf. Fig. 2-11). Hence, injectionThe process of using pressure to force fluids down wells pressures need to be monitored to determine if the changes in pressure may trigger fractureAny break in rock along which no significant movement has occurred. Geological formations with low permeabilityAbility to flow or transmit fluids through a porous solid such as rock and low porosityMeasure for the amount of pore space in a rock require higher injectionThe process of using pressure to force fluids down wells pressures and are thus more susceptible to induced seismicityThe episodic occurrence of natural or man-induced earthquakes.
In addition, mineral precipitation by geochemical interactions between CO2Carbon dioxide, formation waterWater that occurs naturally within the pores of rock formations and reservoirA subsurface body of rock with sufficient porosity and permeability to store and transmit fluids rocks has the potential to significantly decrease formationA body of rock of considerable extent with distinctive characteristics that allow geologists to map, describe, and name it porosityMeasure for the amount of pore space in a rock and permeabilityAbility to flow or transmit fluids through a porous solid such as rock. These changes may result in unexpected (local) pressure build-up and formationA body of rock of considerable extent with distinctive characteristics that allow geologists to map, describe, and name it faulting or fracturing.
Fig. 2-11: Diagram illustrating how injectionThe process of using pressure to force fluids down wells pressures (P) reduce the effective confining and axial strength of a rock formationA body of rock of considerable extent with distinctive characteristics that allow geologists to map, describe, and name it. injectionThe process of using pressure to force fluids down wells pressure counteracts confining and axial pressures, reducing the strength of the rock and causing fractureAny break in rock along which no significant movement has occurred or faulting (Sminchak et al., 20022002 - Joel Sminchak, Neeraj Gupta, C Byrer, P BergmanIssues related to seismic activity induced by the injection of CO2 in deep saline aquiferssee more). |
FormationA body of rock of considerable extent with distinctive characteristics that allow geologists to map, describe, and name it pressure may also influence the stress-strain system. At very high injectionThe process of using pressure to force fluids down wells pressures, rocks may fractureAny break in rock along which no significant movement has occurred in a process termed 'hydraulic fracturing'. Hydraulic fracturing occurs when the injectionThe process of using pressure to force fluids down wells pressure exceeds the intergranular strength of the rock, creating or expanding fractures which may trigger seismic activity.
In addition, the density contrast between formation waterWater that occurs naturally within the pores of rock formations and injected CO2Carbon dioxide may produce a density-driven flow as the lighter, injected fluids migrate upward. Given the large volumes of fluid involved in CO2Carbon dioxide storage(CO2) A process for retaining captured CO2, so that it does not reach the atmosphere operations, the impact of the density contrasts could be capable of influencing stress conditions at depth, thereby causing seismic events (Sminchak et al., 20022002 - Joel Sminchak, Neeraj Gupta, C Byrer, P BergmanIssues related to seismic activity induced by the injection of CO2 in deep saline aquiferssee more).
Risks and potential impacts
InjectionThe process of using pressure to force fluids down wells activities may affect a formationA body of rock of considerable extent with distinctive characteristics that allow geologists to map, describe, and name it far beyond the location of the deep injectionThe process of using pressure to force fluids down wells wellManmade hole drilled into the earth to produce liquids or gases, or to allow the injection of fluids(s). Sometimes, seismic events may occur after injectionThe process of using pressure to force fluids down wells activities are stopped. In addition, earthquakes may be induced in formations wellManmade hole drilled into the earth to produce liquids or gases, or to allow the injection of fluids below the injectionThe process of using pressure to force fluids down wells formationA body of rock of considerable extent with distinctive characteristics that allow geologists to map, describe, and name it. In conclusion, induced microseismicitySmall-scale seismic tremors must be viewed as a manifestation of wider geomechanical deformation (Verdon, 2010) which must be taken into account for riskConcept that denotes the product of the probability of a hazard and the subsequent consequence of the associated event.
Generally, the intensity of induced seismicityThe episodic occurrence of natural or man-induced earthquakes related to CO2Carbon dioxide injectionThe process of using pressure to force fluids down wells is low (Environmental Potection Agency, 2008; Pagnier et al., 20092009 - H. Pagnier, Wildenborg, Nepveu T., M., B. Van der Meer, C. Hofstee, R. Arts, F. Neele, V. Vandeweijer, Ch. Hendriks, R. Brandsma, E. Goetheer, E. Giling, S. Van der Gijp, M. MolagCCS - CO2 Capture and Storage. Report, TNO-Knowledge for businesssee more). The vast majority of induced seismic events does not release enough energy to be felt by people on the surface and the energy from these events can be used for monitoringMeasurement and surveillance activities necessary for ensuring safe and reliable operation of a CGS project (storage integrity), and for estimating emission reductions of process in the reservoirA subsurface body of rock with sufficient porosity and permeability to store and transmit fluids (Myer and Daley, 20112011 - Larry R. Myer, Thomas M. DaleyElements of a best practices approach to induced seismicity in geologic storagesee more). However, they may be precursors to larger events (Mamyer and Daley, 2011). Moderate earthquakes (e.g. of magnitude of 5.1 and 5.2) have already been reported in relation to fluid injectionThe process of using pressure to force fluids down wells activity (Sminchak et al., 20022002 - Joel Sminchak, Neeraj Gupta, C Byrer, P BergmanIssues related to seismic activity induced by the injection of CO2 in deep saline aquiferssee more). None of these, however, were connected with injectionThe process of using pressure to force fluids down wells of CO2Carbon dioxide. The riskConcept that denotes the product of the probability of a hazard and the subsequent consequence of the associated event of inducing seismicityThe episodic occurrence of natural or man-induced earthquakes might be increased when CO2Carbon dioxide is injected into a reservoirA subsurface body of rock with sufficient porosity and permeability to store and transmit fluids in tectonically active regions with high density of active faults (Damen et al., 20062006 - Kay Damen, André Faaij, Wim TurkenburgHealth, Safety and Environmental Risks of Underground CO2 Storage – Overview of Mechanisms and Current Knowledgesee more).
The risks of induced seismicityThe episodic occurrence of natural or man-induced earthquakes can be addressed from a technical perspective through a combination of site characterisation, engineering design, operational procedures and monitoringMeasurement and surveillance activities necessary for ensuring safe and reliable operation of a CGS project (storage integrity), and for estimating emission reductions (Myer and Daley, 20112011 - Larry R. Myer, Thomas M. DaleyElements of a best practices approach to induced seismicity in geologic storagesee more). In particular, the riskConcept that denotes the product of the probability of a hazard and the subsequent consequence of the associated event of induced seismicityThe episodic occurrence of natural or man-induced earthquakes caused by CO2Carbon dioxide storage(CO2) A process for retaining captured CO2, so that it does not reach the atmosphere operation can be minimised by controlling the injectionThe process of using pressure to force fluids down wells pressure (Damen et al., 20062006 - Kay Damen, André Faaij, Wim TurkenburgHealth, Safety and Environmental Risks of Underground CO2 Storage – Overview of Mechanisms and Current Knowledgesee more; Price and Smith, 20082008 - J. Price, B. SmithGeological storage of carbon dioxide - staying safely underground. Bookletsee more). Regulatory limits are imposed on injectionThe process of using pressure to force fluids down wells pressures to avoid significant injectionThe process of using pressure to force fluids down wells-induced seismicityThe episodic occurrence of natural or man-induced earthquakes.
In the early stage of site characterisation, data on the general fault(geology) A surface at which strata are no longer continuous, but are found displaced and fractureAny break in rock along which no significant movement has occurred network geometry in the area will be derived from existing data sources such as wells and seismic surveys. The ideal data set is one from a microseismic network established specifically for each CO2Carbon dioxide storage(CO2) A process for retaining captured CO2, so that it does not reach the atmosphere project (Myer and Daley, 20112011 - Larry R. Myer, Thomas M. DaleyElements of a best practices approach to induced seismicity in geologic storagesee more; Chalaturnyk and Gunter, 20052005 - R. Chalaturnyk and W. D. GunterGeological storage of CO2: Time frames, monitoring and verification in " Greenhouse Gas Control Technologies 7 " Edit by E. S. Rubin, D. W. Keith, C. F. Gilboy, M. Wilson, T. Morris, J. Gale and K. Thambimuthusee more; Benson et al., 20052005 - S. Benson, P. Cook, J. Anderson, S. Bachu, H. Bashir Nimir, B. Basu, J. Bradshaw, G. Deguchi, J. Gale, G. Goerne, W. Heidug, S. Holloway, R. Kamal, D. Keith, P. Lloyd, P. Rocha, B. Senior, J. Thomson, T. Torp, T. Wildenborg, M. Wilson, F. Zarlenga, D. Zhou, M. Celia, Gunter B., J. Ennis King, E. Lindeberg, S. Lombardi, C. Oldenburg, K. Pruess, A. Rigg, S. Stevens, E. Wilson, S. Whittaker, G. Borm, D. Hawkins, A. LeeUnderground geological storage. In: Carbon Dioxide Capture and Storagesee more).